Fluorescence probes for lung carcinoma diagnosis and clinical application
Xiaoyu Zhang【张晓玉】† ab, Feifei Yu【于飞飞】† ad, Zhenkai Wang【王振凯】† ad, Tongmeng Jiang【蒋童蒙】 ad, Xinyu Song 【宋新宇】*c and Fabiao Yu 【于法标】
*ad
aKey Laboratory of Hainan Trauma and Disaster Rescue, The First Affiliated Hospital of Hainan Medical University, Hainan Medical University, Haikou 571199, China. E-mail: yufabiao@hainmc.edu.cn
bDepartment of Pulmonary and Critical Care Medicine, Yantai Affiliated Hospital of Binzhou Medical University, Yantai 264100, China
cState Key Laboratory of Respiratory Disease, Guangzhou Institute of Respiratory Health, National Clinical Research Center for Respiratory Disease, The First Affiliated Hospital of Guangzhou Medicine University, Guangzhou 510120, China. E-mail: songxinyu@stu.gzhmu.edu.cn
dKey Laboratory of Emergency and Trauma, Ministry of Education, Engineering Research Center for Hainan Bio-Smart Materials and Bio-Medical Devices, Key Laboratory of Hainan Functional Materials and Molecular Imaging, College of Emergency and Trauma, Hainan Medical University, Haikou 571199, China
Received 1st February 2023 , Accepted 13th June 2023
First published on 15th June 2023
Abstract
Lung carcinoma is the largest cause of mortality globally, making it the biggest public health issue and a significant barrier to extending human life expectancy. The specific etiology and production process of lung carcinoma are not yet known, and it has a relatively complex and multi-stage occurrence. Early detection and treatment of lung carcinoma can greatly improve the five-year survival rate. Because pre-carcinomatous tumors are small and uncharacteristic in form, early carcinomas are challenging to immediately diagnose with magnetic resonance imaging (MRI), X-ray photography, computed tomography (CT), positron emission tomography (PET), or ultrasonography (US). In order to capitalize on the particular characteristics of carcinoma, methods that can emphasize the molecular distinction between carcinoma and healthy tissue are desperately needed. With the development of bioimaging technology, fluorescent probes present a potential solution to this clinical problem. Fluorescent probes have been considered to be effective chemistry tools for achieving early detection and identification of tumor lesions, because molecular imaging technology can explicitly illustrate lesion boundary information during surgery. Fluorescent probes can also qualitatively and quantitatively analyze the lesion state at the cellular and molecular levels in the living body. This review will cover and provide an overview of the most recent developments in fluorescence probe technology for the accurate detection and clinical therapy of lung carcinoma. We anticipate that this review will serve as a spark for the development of intelligent molecular fluorescent probes for lung carcinoma clinical image-guided surgery and imaging diagnosis.
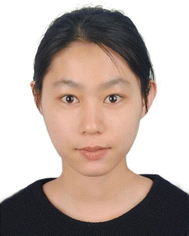
Xiaoyu Zhang | Xiaoyu Zhang received her Master's degree in Clinical Medicine from Binzhou Medical University in 2018. Currently, she is working as a doctor at the Department of Pulmonary and Critical Care Medicine, Yantai Affiliated Hospital of Binzhou Medical University. Her main research field is the imaging of small molecular fluorescence probes for lung diseases. |
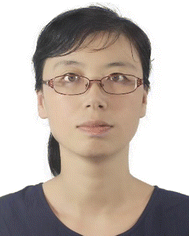
Feifei Yu | Feifei Yu received her Bachelor's degree in Applied Chemistry from Shandong Normal University in 2006. Then she works as a laboratory technician at Hainan Medical University now. Her main research areas are marine drug analysis and design and synthesis of fluorescent probes. |
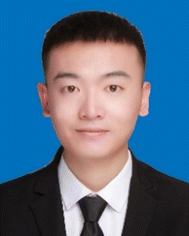
Zhenkai Wang | Zhenkai Wang received his Master's degree in Clinical Medicine from Binzhou Medical University in 2020. Now he is a PhD candidate at The First Affiliated Hospital of Hainan Medical University, Hainan Medical University. His main research direction is the design and application of small molecule fluorescent probes for clinical medical detection. |
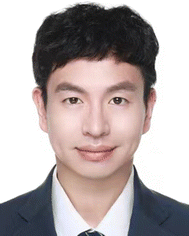
Tongmeng Jiang | Tongmeng Jiang received his Bachelor of Medicine and Ph.D. in Surgery from Guangxi Medical University in 2018. He finished his postdoc at the School of Materials Science and Engineering, Zhejiang University, in May 2022, and then he joined Hainan Medical University as an associate professor. His research focuses on biomaterials, tissue regeneration, translational medicine, imaging and oncology. |
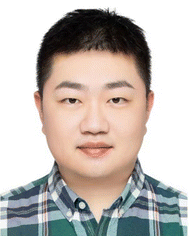
Xinyu Song | Xinyu Song received his Doctor's degree in Internal Medicine from Guangzhou Medical University in 2021. He is now serving for Guangzhou Institute of Respiratory Health, The First Affiliated Hospital of Guangzhou Medicine University as a respiratory physician. His current interests are development of fluorescence bioimaging and theranostic tools. |
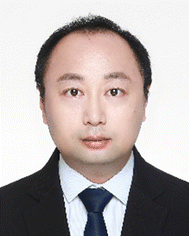
Fabiao Yu | Prof. Fabiao Yu received his Ph.D. degree from Dalian University of Technology and Dalian Institute of Chemical Physics, Chinese Academy of Sciences in 2013. After four years' work at Yantai Institute of Coastal Zone Research, Chinese Academy of Sciences as an associate professor, he joined Hainan Medical University from 2018 as a full professor. His research interests focus on molecular diagnosis of tropical diseases, multi-modal visual surgery navigation, and point-of-care testing (POCT) technology. |
1. Introduction
The near-infrared (NIR) fluorescence imaging technology is an active and non-invasive molecular imaging method.1 The rapid development and improvement of near-infrared fluorescent probes have improved some optical properties, such as hydrophilicity, stability, and high quantum yields.2 These features can greatly strengthen the detection selectivity and sensitivity. An excellent NIR imaging probe requires excellent chemical and photophysical properties. The spectrum of the NIR fluorescent reagent should be in the NIR range, i.e., 650–950 nm (near-infrared I region), 1000–1350 nm (near-infrared II region), and 1500–1800 nm (near-infrared NIR III region)3 to reduce the interference from the autofluorescence and increase the tissue penetration depth. Many NIR fluorophores exhibit excellent properties for selective accumulation in tissues, organs, or tumor lesions in vivo, and enhance tumor sites by binding to tumor-specific molecular biomarkers such as proteases, peptides, and antibodies.2,4–7 NIR probes that possess specificity and sensitivity toward tumor sites have been widely utilized in tumor-targeted imaging and tumor treatment.8 These probes indicate unique optical and biomedical imaging properties for broad development prospects.
Lung carcinoma displays the highest morbidity and mortality among various malignant tumors.9 The complete resection of the lesion site, the negative margin, and clean of metastatic lymph nodes determine the prognosis of carcinoma patients.10–12 Lung carcinoma is still a challenging problem.13–23 It is expected that the development of fluorescent probes can serve the clinical work well, so the clinical application strategy of fluorescence-guided surgery (FGS) can be realized. During the surgical procedure, the fluorescent probes are used for the specific visual imaging of the lesion site to detect and discriminate malignant and normal tissues, which can reduce the surgical trauma and improve the prognosis of patients.24,25 Compared with other traditional medical imaging models, such as magnetic resonance imaging (MRI), X-ray photography, computed tomography (CT), positron emission tomography (PET), or ultrasonography (US), FGS has its own unique safe, effective, and real-time technique for lung carcinoma surgery.26 Near-infrared fluorescence imaging to guide surgery is conducive to the development of clinical medicine. Also, fluorescence imaging has breathed new life into surgical oncology and is expected to have a considerably essential impact on personalized oncology in the future. In this review, we will dedicatedly focus on fluorescent probes and the specific response methods for the potential application of lung carcinoma diagnosis in clinical. We also exclusively highlight near-infrared probes for fluorescence-navigation surgery and the clinical application of near-infrared tumor-targeted probes in lung surgery.
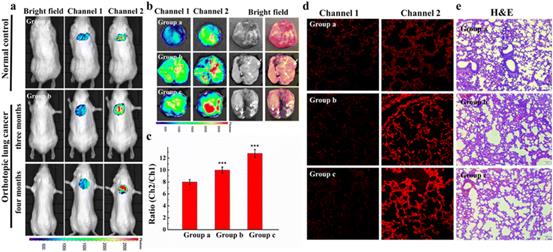
|
| Fig. 7 Small animal fluorescence imaging of Cys in orthotopic lung carcinoma mice. a) Probe images of Cys in mice. b) Ex vivo fluorescence imaging of lung carcinoma. c) The average ratio intensity values of mouse groups a–c within 30 min. Ratio values in c). d) Confocal fluorescence images of fresh lung tissue slices in mouse groups a–c. e) H&E stained lung tissues from normal tissues and carcinoma lesions in groups a–c. Reproduced with permission from ref. 59, Copyright 2020 Elsevier. |
|
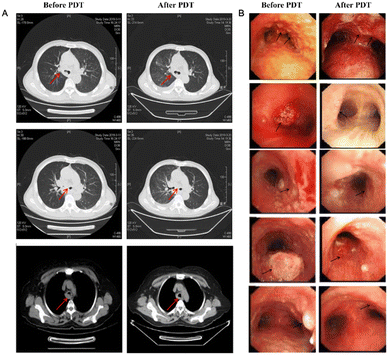
|
| Fig. 8 (A) CT image changes in the chest after PDT treatment 3 months later. (B) CT image changes in bronchoscopy after PDT treatment 3 months later. Reproduced with permission from ref. 70, Copyright 2020 Spring Nature. |
|
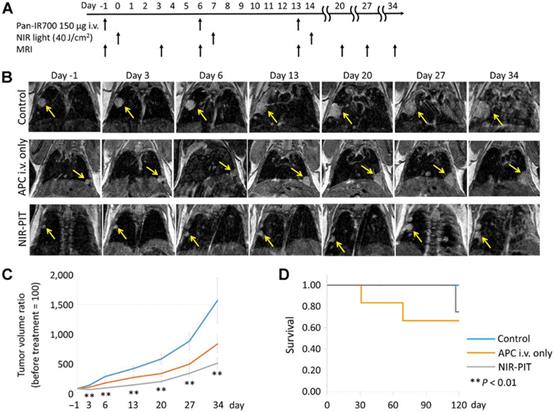
|
| Fig. 10 In vivo effects of probe NIR-PIT on the mouse model of lung tumor. (A) NIR-PIT regimen of APC administration and exposure to near-infrared light. MIR images were acquired at the corresponding time points. (B) MIR images of the lung tumor bearing mouse model. The measured size of the lung tumors of the animals developed rapidly in the control group and APC i.v. only groups, whereas the measured size of the lung tumors developed only gradually in the NIR-PIT group. (C) The tumor volume ratio was suppressed considerably in the NIR-PIT group compared to the control group, while there was no obvious discrimination in the tumor volume ratio in the APC i.v. only group compared to the control group. (D) The experimental results showed that there was no evident difference in the survival rate of the three groups of mice. Reproduced with permission from ref. 77, Copyright 2017 American Association for Cancer Research. |
|
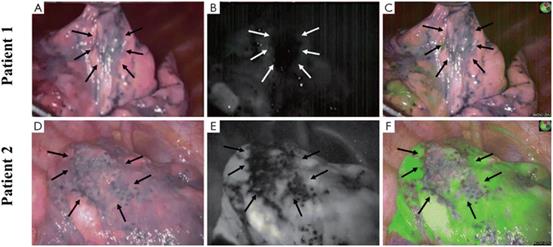
|
| Fig. 12 Bullous lesions illustrated using a near-infrared thoracoscope. (A) The image of bullous lesion under white light; (B) the image of bullous lesion for fluorescence emission with 0.2 mg kg−1 ICG; (C) the syncretic mode of bullous lesion; (D) the image of bullous lesion under white light; (E) the image of bullous lesion for fluorescence emission with 0.6 mg kg−1 ICG; (F) the syncretic mode near bullous lesion. Reproduced with permission from ref. 120, Copyright 2016 Journal of Thoracic Disease. |
|
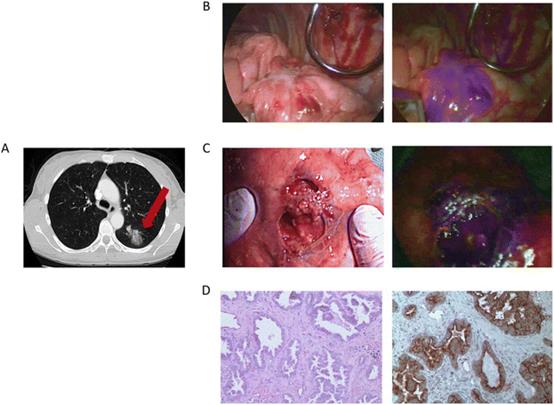
|
| Fig. 13 The clinical case was a 55-year-old female. (A) Preoperative chest imaging revealed a mass in the left lower lobe; (B) white light navigation image and near-infrared fluorescence navigation image of the lesion obtained during surgery; (C) white light navigation image and near-infrared fluorescence navigation image of the lesion after operation; (D) pathological section verified an invasive adenocarcinoma. H&E staining and immunohistochemistry illustrated a strong folate receptor staining. Reproduced with permission from ref. 123, Copyright 2016 American Cancer Society. |
|
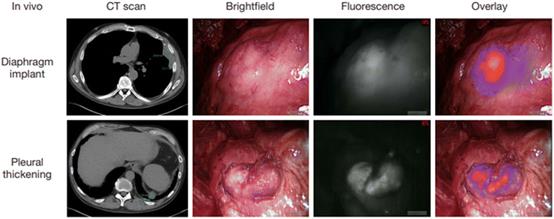
|
| Fig. 14 Intraoperative near-infrared images for two mesothelioma representative patients. Preoperative CT scan, thoracoscopic view in vivo, fluorescence images, and merge of fluorescence images and brightfield images are shown from left to right. Reproduced with permission from ref. 128, Copyright 2017 Translational Lung Cancer Research. |
|
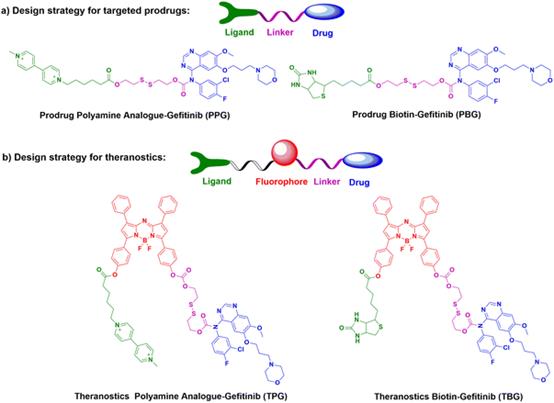
|
| Fig. 15 The design strategies and chemical structures of part a) potential prodrugs PPG & PBG, and part b) theranostics TPG & TBG. |
|
|
|
|
|
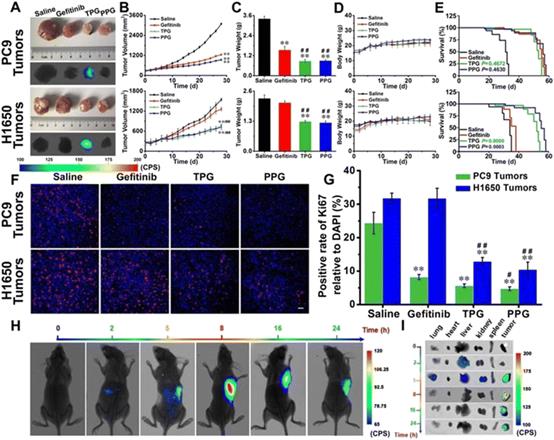
|
| Fig. 16 Efficacy of fluorescence navigation in living mice. Thymus deficient mice bearing PC9 cells and H1650 cells were incubated with saline, gefitinib, TPG, and PPG, respectively. (A) The dissected solid tumors and their fluorescence images. (B) Tumor mass sizes. (C) Tumor mass weights. (D) Mouse body weights. (E) Survival curves. (F) Immunofluorescence staining of Ki67. (G) Statistical analysis derived from (F). (H) Fluorescence images of the mice at different time points after tail vein injection of TPG. (I) Fluorescence images of the dissected organs lung, heart, liver, kidney, spleen, and tumor masses from (G). Reproduced with permission from ref. 129, Copyright Ivyspring International Publisher. |
|
Conclusions and perspective
Fluorescence bioimaging technology has become a promising chemical tool for biologists and physicians to investigate biological events because they can supply visual information with high spatial and temporal resolution in situ and in real time. Intraoperative molecular fluorescence imaging provides accurate tumor removal and the ability to determine whether tissue margins are still present while providing objective, real-time tumor visualization during surgical procedures. Compared with traditional probes with UV-visible emission wavelengths, near-infrared fluorescent probes have unique advantages, such as near-infrared emission which can prevent the interference of native biomolecular autofluorescence and achieve higher signal-to-noise ratios. The longer wavelengths of near-infrared fluorescent probes are able to acquire images in deeper tissue. Moreover, the near-infrared excitation and emission exhibit less damage to biological samples than UV-visible wavelengths of light. Over the past decade or so, near-infrared fluorescent probes have been developed to detect various biological species, monitor drug delivery, and perform surgical navigation in vivo and in vitro, but current tumor-targeted fluorescent probe libraries cannot identify all carcinomas, thus requiring the design of new tumor-specific fluorescent dyes used for carcinomas that are currently undetectable.
The early diagnosis and precise treatment of lung cancer are crucial for reducing patient mortality and improving the patient survival rate. Fluorescent probes for lung cancer are of importance in the clinical diagnosis and treatment. For diagnosis, the fluorescent probe will brighten the lung cancer tissue, allowing the operator to locate cancerous tissue surrounding healthy tissue with fluorescence guided endoscopy or percutaneous lung biopsy. The failure rate of biopsy and bleeding complications thereby decrease. For treatment, fluorescence tracing can aid to identify the risk of the tumor edge and distant metastasis for guiding accurate tumor resection, reducing the risk of tumor recurrence and metastasis, and preserving the patient's healthy lung tissue. Therefore, probes with good biocompatibility, high fluorescence intensity, and accurate targeting will benefit more clinical patients.
Fluorescence-guided surgery (FGS) is specifically targeted to and accumulates in tumors for molecular imaging, a technology capable of improving carcinoma patient outcomes by offering smart and non-invasive diagnosis, reducing total surgical time, and enabling more complete tumor resection. We believe that the ability to utilize new types of near-infrared fluorescent probes for intraoperative optical navigation can provide more sensitive and accurate strategies for carcinoma resection guidance, resulting in better surgical prognosis. However, in the process of research, it is also found that some fluorescent probes are only passively accumulated and cannot be used to distinguish tumors with inflammation. In the future, more precise targeting reagents need to be developed to solve this problem.