细胞外囊泡(EVs)是包裹在脂膜中的纳米大小的囊泡,几乎所有类型的细胞都能释放。EVs具有运输生物分子进行细胞间通讯的生理功能,被认为是诊断和有效药物载体的有价值的生物标志物。
近期,来自海南医学院的于法标团队发表了综述性评论,重点介绍了EVs荧光标记策略的最新进展及其在生物医学应用和活体生物学研究中的应用以及单个EV成像的进展。最后,讨论了研究EVs生理特性和进一步生物医学应用方面的挑战和未来的前景。相关内容以“Fluorescence labeling of extracellular vesicles for diverse bio-applications in vitro and in vivo”为题在线发表在Chemical Communications(IF: 6.065)杂志上 (doi: 10.1039/d3cc00998j)。Fluorescence labeling of extracellular vesicles for diverse bio-applications in vitro and in vivo
Received 28th February 2023 , Accepted 2nd May 2023
First published on 3rd May 2023
DOI: 10.1039/D3CC00998J (Highlight) Chem. Commun., 2023, 59, 6609-6626
https://pubs.rsc.org/en/content/articlelanding/2023/cc/d3cc00998j
Abstract
Extracellular vesicles (EVs) are nanosized vesicles enclosed in a lipid membrane that are sustainably released by nearly all cell types. EVs have been deemed as valuable biomarkers for diagnostics and effective drug carriers, owing to the physiological function of transporting biomolecules for intercellular communication. To investigate their biological properties, efficient labeling strategies have been constructed for EV research, among which fluorescence labeling exerts a powerful function due to the capability of visualizing the nanovesicles with high sensitivity both in vitro and in vivo. In one aspect, with the help of functional fluorescence tags, EVs could be differentiated and categorized in vitro by various analytical techniques, which exert vital roles in disease diagnosis, prognosis, and treatment monitoring. Additionally, innovative EV reporters have been utilized for visualizing EVs, in combination with powerful microscopy techniques, which provide potential tools for investigating the dynamic events of EV release and intercellular communication in suitable animal models. In this feature article, we survey the latest advances regarding EV fluorescence labeling strategies and their application in biomedical application and in vivo biology investigation, highlighting the progresses in individual EV imaging. Finally, the challenges and future perspectives in unravelling EV physiological properties and further biomedical application are discussed.
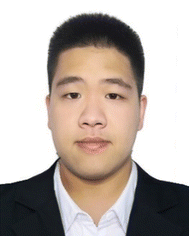 Yun He | Yun He is currently a master's student majoring in clinical diagnostics in Hainan Medical University. He received his bachelor's degree in clinical medicine from Nanchang University in 2021. His current research focuses on the fluorescence-based detection of extracellular vesicles for accurate diagnosis of various diseases. |
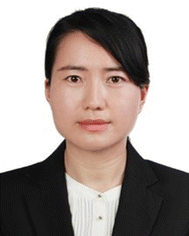 Yanlong Xing | Yanlong Xing received her PhD degree from Technical University of Berlin (Jan 2017) and was a doctoral researcher in the Swiss Federal Institute of Technology (2013–2015) and Postdoctoral Research Fellow in ISAS Berlin (2017–2018). She is now an associate professor at Hainan Medical University. Her current research focuses on extracellular vesicle-based liquid biopsy and microfluidic-assisted detection of biomolecules using fluorescence and Raman microscopy. |
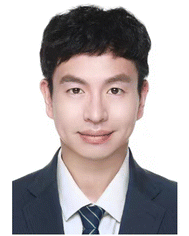 Tongmeng Jiang | Tongmeng Jiang received his PhD degree in Surgery from Guangxi Medical University in 2018. After finishing his postdoc at the School of Materials Science and Engineering, Zhejiang University, he joined Hainan Medical University as an associate professor in June 2022. His research focuses on tissue regeneration, translational medicine, imaging, oncology, and related chemistry and engineering. |
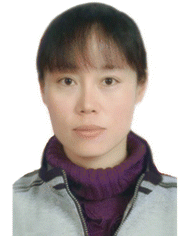 Juan Wang | Juan Wang received her PhD in biomedical engineering for Tongji University in 2019. Currently, she is an associate professor in the College of Basic Medicine and Life Sciences at Hainan Medical University. Her research focuses on the research of stem cell treatment technology. |
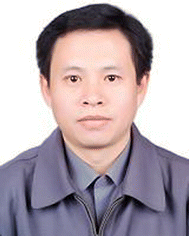 Shenggang Sang | Shenggang Sang received his MS degree from Lanzhou University in June 2007 and has been working at the First Affiliated Hospital of Hainan Medical University since September 2007. He was awarded Hainan Leading Talent and Nanhai Talent. He has published 10 professional books and more than 30 research articles. His research focuses on the application of immune cells in the clinical therapy of diseases. |
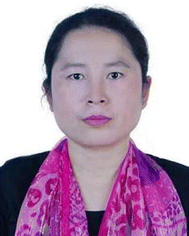 Hong Rong | Hong Rong received her bachelor's degree from Hainan Medical University in clinical nursing. She has gained 15 years’ experience in clinical nursing practice at the People's Hospital of Heze before joining the First Affiliated Hospital of Hainan Medical University in 2008. She is now working as a senior nurse and participates in the clinical trial programs of the university. She has worked as a main participant in several national and provincial research projects. |
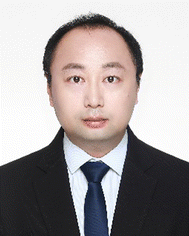 Fabiao Yu | Prof. Fabiao Yu received his PhD degree from Dalian University of Technology and Dalian Institute of Chemical Physics, Chinese Academy of Sciences in 2013. After four years’ work at Yantai Institute of Coastal Zone Research, Chinese Academy of Sciences, as an associate professor, he joined Hainan Medical University from 2018 as a full professor. His research interests focus on molecular diagnosis of tropical disease, multi-modal visual surgery navigation, and point-of-care testing (POCT) technology. |
为了研究EV的生物学特性,人们已经建立了众多有效的标记策略用于其研究,其中荧光标记由于能够在体内外高灵敏度地可视化纳米级囊泡而发挥了强大的作用。一方面,在功能荧光标记的帮助下,EVs可以通过各种分析技术在体外进行区分和分类,这在疾病的诊断、预后和治疗监测中发挥着重要作用。另一方面,新型的EVs报告分子已被用于可视化EVs,结合强大的显微镜技术,为在合适的动物模型中研究EVs释放和细胞间通讯的动态事件提供了极具潜力的工具。
为了实现EVs的高分辨率成像,有效的标记策略是使其能够在亚细胞水平上可视化不可或缺的技术。根据荧光报告分子与EVs结合的方式,通常可分为直接荧光标记法和间接荧光标记法(图2)。前者主要是通过与抗体/适配子结合或生物正交引入荧光标记来进行体外分析。后者直接标记EVs主要是用于体内分析。各种成像技术与创新的标记策略相结合,为在单囊泡水平上研究EVs的生物学提供了极好的工具。
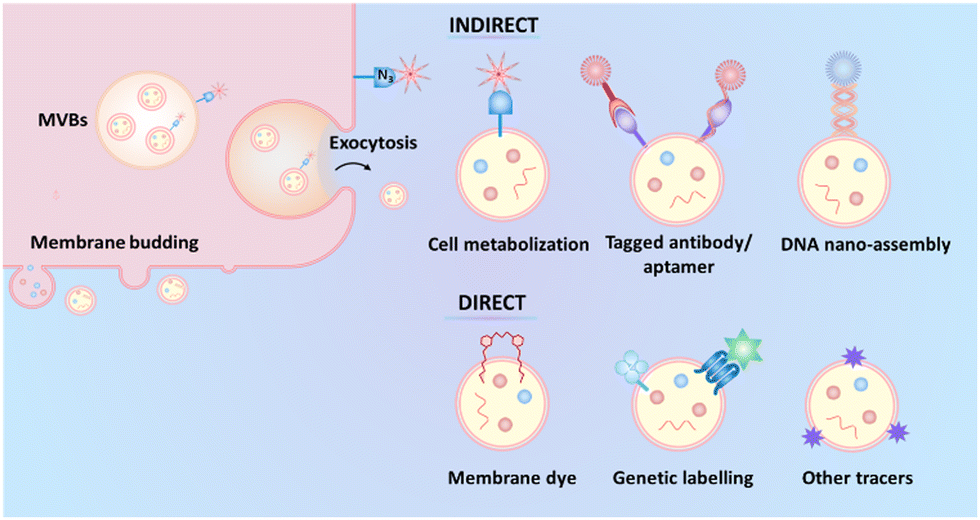
Fig. 2 Schematic illustration of EVs various indirect and direct fluorescent labeling strategies.荧光标记EVs将在多个领域发挥巨大的应用潜力,包括开发先进的分析技术,生物医学应用包括诊断和治疗(主要通过体外分析),以及生物学研究(活细胞和体内的生物成像)等。理想情况下,这些方案对EVs定性和定量分析具有足够的分辨率和灵敏度。此外,荧光标记EVs的体外分析可以通过区分来自患者和健康对照的EVs,促进EVs相关生物标志物的发现,并有助于疾病的诊断。随着标记策略和检测技术的发展,可以有效提高诊断的准确性,从而体现出了这些技术在临床转译中的巨大潜力。另一方面,对EVs生物学的深入了解对于广泛开发这些囊泡的临床价值至关重要。在研究EVs的生物学特性时,活体细胞模型中的生物成像在一定程度上揭示了EVs的生物学行为,包括EVs的生物发生、分泌、转运、内吞等。为了揭示EVs在体内的生物成像,需要应用合适的3D模型系统。到目前为止,黑腹果蝇、线虫,小鼠和斑马鱼已被用作EVs生物学研究的活体模型。该综述中重点介绍了EVs体内生物成像的最新进展,以揭示EVs的生物学,包括EV的生物发生和运输、囊泡-细胞通讯、生物分布、疾病进展和治疗。为了揭示体外和体内EVs生物学的多样化应用,未来需要考虑几个关键点。首先,需要先进的单个EV分析技术来揭示不同EVs亚群的异质性。单EV分析的进展不仅有助于加深对EVs生物学的认识,而且有助于将EVs作为疾病诊断的生物标志物应用于生物医学领域。其次,在EVs生物学研究方面,需要进一步研究EVs在细菌和病原体中的生物学,并与活细胞和各种生物模型中的EVs生物学进行比较。第三,尽管在囊泡-细胞通讯中已经揭示了几点,但完整地强调调节EV功能的分子机制仍然具有挑战性。在解决成像方面的技术挑战和分子生物学中的其他问题方面仍需要进一步的努力。因此,进一步发展荧光标记与其他先进的成像方法之间的合作是未来EVs体外和体内生物学特性研究的趋势。总之,EVs生物学的知识增长迅速,这为生物医学提供了巨大的前景。基于分子生物学、生物成像、工程学等多学科技术,EVs在诊断和治疗方面的临床转译具有广阔的前景。Table 1 Comparison of various direct labeling strategies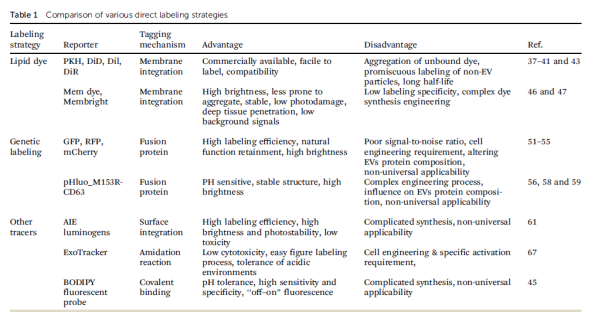
Fig. 3 (A) (i) Visualisation of the appearance individual fluorescent spots within the SSC (low)eGFP(+) sEV population with different brightness and size/pixel coverage. Reproduced from ref. 69 with permission of Taylor & Francis Group of 2019. (ii) Multiple phenotyping of the gated sEV (G1) is proved by EV labeling, fluorescent labeling of CD9, CD63, and CD81 binding (PE, Ch03) and HLA-DR (PECy7, Ch06). Reproduced from ref. 70 with permission of Frontiers of 2018. (B) (i) Schematic illustration of lab constructed HSFCM. (ii) Immunostaining with PE-conjugated anti-CD147 and a bivariate dotplot of the PE orange fluorescence versus SSC for EVs derived from patient samples. (iii) Plasma granule levels of CD147-positive EVs in healthy controls (n = 32) and colorectal cancer patients (n = 37) (mean ± s.d.). The P-value was calculated using ANOVA test. Reproduced from ref. 74 with permission of American Chemical Society of 2018. (C) (i) Representative image of the mammaglobin-a population observed on the Nano-FCM. (ii) Quantitatively detect the concentration of mammaglobin-a in plasma samples of healthy (50), benign (56), and breast cancer (219) by nano-FCM. Reproduced from ref. 82 with permission of Royal Society of Chemistry of 2021.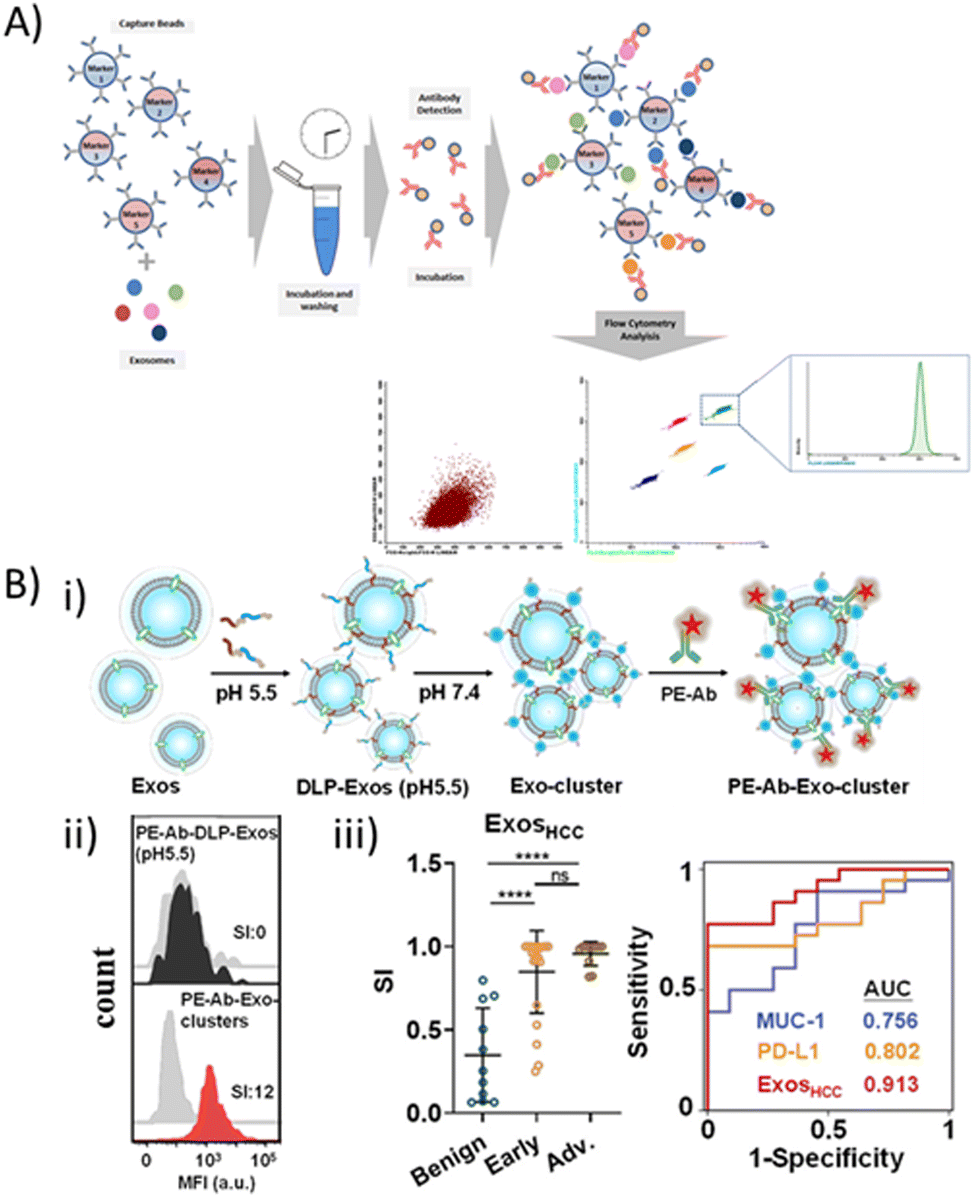
Fig. 4 (A) Bead-based Array Principle. Detection antibodies fluorescently labelled, usually in the most sensitive channel PE (FL2) for each bead population, the PE signal is then quantified using a flow cytometer. Reproduced from ref. 85 with permission of Elsevier of 2019. (B) pH mediated EVs assembled into clusters for FCM analysis. (i) Schematic illustration of Exo-cluster preparation and immunolabeling with fluorescent antibodies (PE-Ab). (ii) FCM results for DLP-Exos at pH 5.5 and clusters before (light gray) and after (gray, black, and red) immunolabeling with PE-Ab (e.g., CD63). (iii) Scattering plots of the ExoHHC levels in the plasma of controls, early-stage (stage A) and advanced-stage (stage B–D) HCC patients. (E) ROC analysis showing the diagnostic ability of sEV markers and ExoHHC for early diagnosis. ExosHCC shows 77.3% sensitivity and 100% specificity. Reproduced from ref. 89 with permission of American Chemical Society of 2021.
Fig. 5 (A) Scheme of HOLMES-ExoPD-L1 analysis. (B) Analysis of the binding performance of the MJ5C aptamer and PD-L1 antibody to PD-L1 positive EVs conjugated beads by FCM and confocal images. (C) Circulating exoPD-L1 detected by HOLMES-ExoPD-L1. (i) Healthy control (n = 22) and cancer patients (n = 34). (ii) Cancer patients with different stages of adenocarcinoma (8 cases in stage I, 16 cases in stage II, 10 cases in stage III & IV). (iii) Patients with localized (n = 21) and metastatic (n = 13) cancer. Reproduced from ref. 32 with permission of John Wiley and Sons of 2020.
Fig. 6 (A) (i) Working principle of the single-vesicle fluorescence imaging with TIRFM. (ii) TIRFM images of miR-21-EVs from clinical serum samples from cancer patients and healthy controls. Scale bar = 3 μm. (iii) Fluorescence intensity analysis of miR-21-EVs at the single-vesicle level in different clinical serum samples. Reproduced from ref. 36 with permission of Ivy spring of 2019. (B) (i) Representative imaging of EV membrane protein (top: wide-field TIRFM image; lower: super-resolution image). Scale bar: 2 μm. (ii) EVs ϒ value of four biomarkers in pancreatic and breast cancer samples. (iii) Results of detection of 7 unknown samples using the generalized model. Reproduced from ref. 35 with permission of John Wiley and Sons of 2019.
Fig. 7 (A) CD63 and CD9 transiently co-localize in MVB and at the PM. (i) Live imaging of HeLa cells co-transfected with the CD63-mCherry and CD9-eGFP RUSH plasmids (z-projection). White arrows show EVs with CD63 and CD9 co-localization. Scale bar: 5 μm. (ii) Confocal imaging of co-transfected HeLa cells (CD63, CD9 & Rab7, z-projection). Scale bar: 10 μm. Mander's coefficients represent the properties of various EVs. Reproduced from ref. 101 with permission of Springer Nature of 2021. (B) Imaging of intracellular precursor EVs using a leuco-BODIPY probe in HeLa cells that express GFP-CD81 (green) and BODIPY (red). R values indicate the Pearson colocalization coefficients. Scale bar = 10 μm. Reproduced from ref. 108 with permission of American Chemical Society of 2021. (C) Visualization of EV release in MDA-MB-231 tumor sections in vivo. Arrows point to EVs of different sized. Scale bars: 10 mm, 1 mm and 1 mm. Reproduced from ref. 49 with permission of Elsevier of 2015.
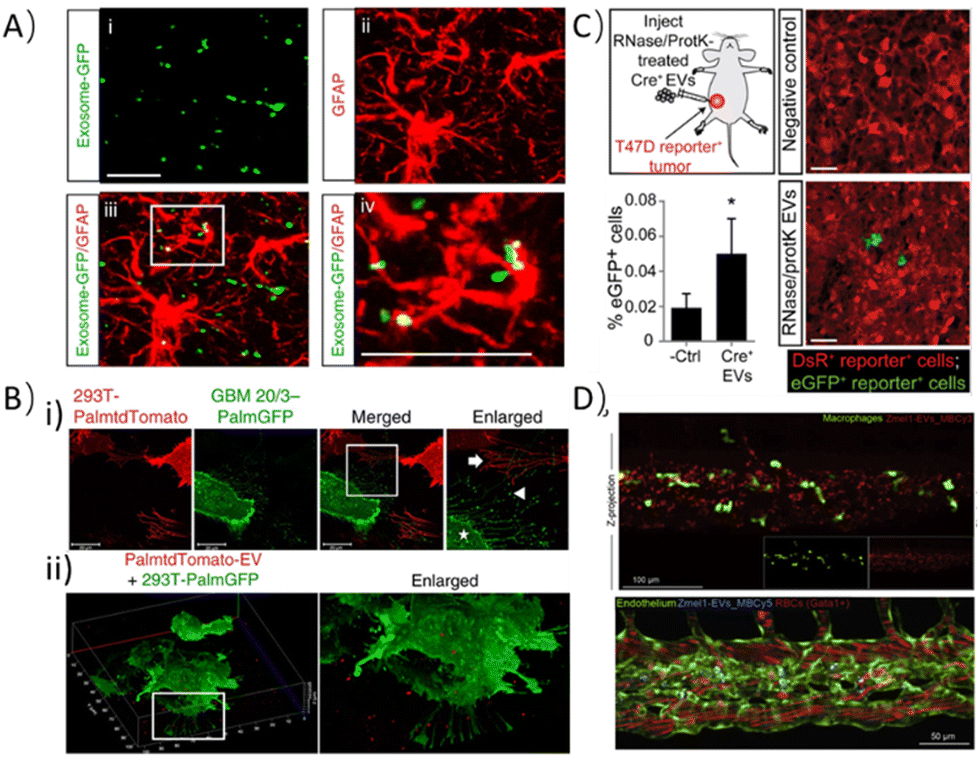
Fig. 8 (A) Representative bioimaging of the internalization of neuron-released EVs (CD63-GFP+ puncta) into GFAP+ astrocytes in AAV8-CaMKII-Cre_mCherry virus injected CD63-GFPf/+ mice. (i) CD63-GFP fluorescence; (ii) GFAP immunostaining; (iii) merge of (i) and (ii); (iv) magnification view of (iii); scale bar: 50 μm. Reproduced from ref. 113 with permission of Springer Nature of 2019. (B) Live imaging of exchange and uptake of EV. (i) 293T-PalmtdTomato and primary GBM-PalmGFP cells were co-cultured and imaged. (ii) 3D Z-stack images displaying PalmtdTomato EVs incubated in 293T-PalmGFP cells for 1.5 h. Reproduced from ref. 50 with permission of Springer Nature of 2015. (C) The transfer of EVs between tumor cells in living mice. Representative images of Cre+ MDA-MB-231 tumors and quantification of eGFP+ cells (n = 3 mice). Scale bars = 50 mm. Reproduced from ref. 49 with permission of Elsevier of 2015. (D) EVs derived from Zmel1 are mostly internalized by macrophages and endothelial cells. Confocal images of MemBright-Cy3 labeled Zmel1 EVs in Tg(mpeg1: GFP) zebrafish embryo (upper, macrophage and lower, endothelium). Reproduced from ref. 48 with permission of Elsevier of 2019.
Fig. 9 (A) In situ monitoring of EVs derived from breast cancer cell in mice after administration of fluorescently labeled MCF-7-, MDA-MB-231-, and HS578T-derived EVs. Reproduced from ref. 23 with permission of American Chemical Society of 2021. (B) In vivo tracking of injected EVs. The FMT imaging of the Z plane displays elevated fluorescence response mainly at the spleen and liver in Alexa680-EVGlucB and not in the control model. Reproduced from ref. 116 with permission of American Chemical Society of 2014. (C) Fluorescent-labeled EVs obtained from shControl, shRalA or shRalB cells were equally injected intravenously and the lung accumulation 1 h post-injection was studied. Representative ex vivo images and quantification data displaying the overall lung fluorescence. Each dot indicates one mouse. Reproduced from ref. 119 with permission of eLife Sciences of 2021. (D) Melanoma EVs Promote Tumor Growth in Zebrafish. In Tg(Fli1:GFP) embryos, Zmel1-tdTomato tumor growth is promoted after injection of Zmel1 EVs. Reproduced from ref. 48 with permission of Elsevier of 2019.Perspectives and conclusions
Fluorescence labeling technology has matured, enabling researchers to gain deep insights into the EV properties in vitro and in vivo, with the assistance of advanced microscopy techniques. Various fluorescent probes provide novel methods to investigate the properties of EV and its subpopulations. In vitro technologies can be utilized for the qualitative and quantitative analysis of EVs with enough resolution and sensitivity, thus, promoting the discovery of EV-related biomarkers and facilitating liquid biopsy of diagnosing various diseases including cancer. The in vivo biodistribution of EVs provides not only the roles of cell–cell communication, but also more detailed information into their biogenesis, trafficking, and secretion in the biological milieu. Knowing the EV behaviour in vivo, including the biogenesis, organ distribution also provides evidence for monitoring innate indicators of pathological and physiological processes, and promotes the biomedical application such as markers for diagnosis and prognosis, and therapeutic targets.
To reveal the diverse biomedical application in vitro and EV biology in vivo, several critical points need to be taken into consideration in the future. Firstly, advanced single EV analysis techniques are highly demanded to disclose the heterogeneity of different EV subpopulations. The progress in single EV analysis could not only promote the understanding of EV biology, but also improve biomedical application of EVs as biomarkers for promising disease diagnosis. Secondly, for EV biology investigation, the EV biology in bacteria and pathogens need to be further investigated and compared with that in living cells and various organism models. In particular, how bacteria and pathogens affect the disease state of organisms through released EVs need to be studied. Thirdly, although several points have been revealed in vesicle–cell communication, complete underlining the molecular mechanisms that regulate EV function remain challenging.
Further efforts are still required in solving the technical challenges in imaging and other issues in molecular biology. Therefore, further development of important synergies between fluorescence labels and other advance imaging methods such as FCM, confocal microscopy, SRM, TIRF is the trend of future investigation of biological properties of EV in vitro and vivo. In conclusion, knowledge on EV biology has grown rapidly, which offers tremendous prospects for biomedicine. Based on the multi-disciplinary technologies of molecular biology, bioimaging, engineering etc., a bright future for clinical translation of EVs in diagnostics and therapeutics can be envisioned.